Science
Related: About this forumRecord High Magnetic Field (45.5 Tesla) Achieved Using High Temperature Superconducting Ceramic.
The paper I'll discuss in this post is this: 45.5-tesla direct-current magnetic field generated with a high-temperature superconducting magnet (David C. Larbalestier et al Nature, Published On Line 6/12/2019).
Recently I met with an old friend and his wife for dinner, who I hadn't seen for a long time, and we got around, of course, as old friends do when they get old, to talking about our kids, and when I discussed what my kid is doing over the summer we got on the subject of neutrons. I think about all the time about neutrons, albeit in a very different way than my son thinks about them, since my son is concerned with their De Broglie wavelengths and I personally am not at that level of detail with neutrons and couldn't care less (effectively) about neutron wavelengths (although probably I should). Anyway, I told my friend that my kid would be working with neutron beams, which generated the question from my friend, chemist to chemist (since we think about mass spec), "How can you focus neutrons? They have no charge."
I answered, "You can't."
A short time afterwards my kid and I were chatting on the phone and he told me all about the "big" magnet they have in the lab where he's working, which is a 14 Tesla magnet and we got to talking and he reminded me that neutrons have a magnetic moment (thus proving that they cannot truly be elementary particles).
So there's that.
Anyway, in my lazy head, I kind of thought that the upper limit for magnets was in the neighborhood of nine or ten Tesla, and I was impressed to hear that I was wrong, but I had no idea of how wrong I was.
I recall the first time I saw a "high temperature" super-conducting material; it was at an ACS local section meeting in San Diego where the speaker, whose name I've forgotten, brought a Dewar of liquid nitrogen (the "high temperature" as compared to liquid helium in most superconducting magnet systems) and some tiny little disks. He soaked the disks in liquid nitrogen, applied a current to them and did some levitation experiments using a strong conventional magnet.
At that time in my life, I was inclined to have unrealistic fantasies of the type where wind turbines in North Dakota powered Southern California using superconducting power lines.
The rap on this lanthanide/copper oxide "high temperature" superconductors was #1 that they could only carry a low density current before breaking down and #2, that they were ceramics and could not be drawn into wire.
Scratch that, well, maybe not a wire, but a tape...
From the (open sourced) abstract:
...and some more from the text of the paper...
The coil was the third in a series of LBCs, with LBC1 reaching 40 T, LBC2 attaining 42.5 T and LBC3 achieving 45.5 T, all in the same 31.1-T background field and all with nominally the same design. We found that tests in liquid nitrogen were valuable for checking joint resistances and establishing key operation parameters, including the coil constant, the characteristic coil resistance, Rc, and the charging time constant12,13,14,15. Occasionally some joints were remade to reduce resistive losses and minimize coil warming during test. For the high-field test, LBC3 was placed in a 37-mm-diameter liquid helium cryostat inside the resistive background magnet (50-mm warm bore, 18 MW, 31.1 T) at the National High Magnetic Field Laboratory (MagLab). To mitigate trapped helium bubbles, which allow heating of the magnet above 4.2 K during charging16, a small-diameter tube above the magnet periodically pumped away helium vapour during the test so as to limit the temperature of the top surface of LBC3, which reached 7 K at the moment of the 45.5-T quench. A Hall sensor calibrated up to 44.8 T in the 45-T hybrid magnet was used to measure the centre field, together with a pickup coil (the linearity of which was confirmed in multiple charging tests before the main 45.5-T test).
Some pictures from the paper:
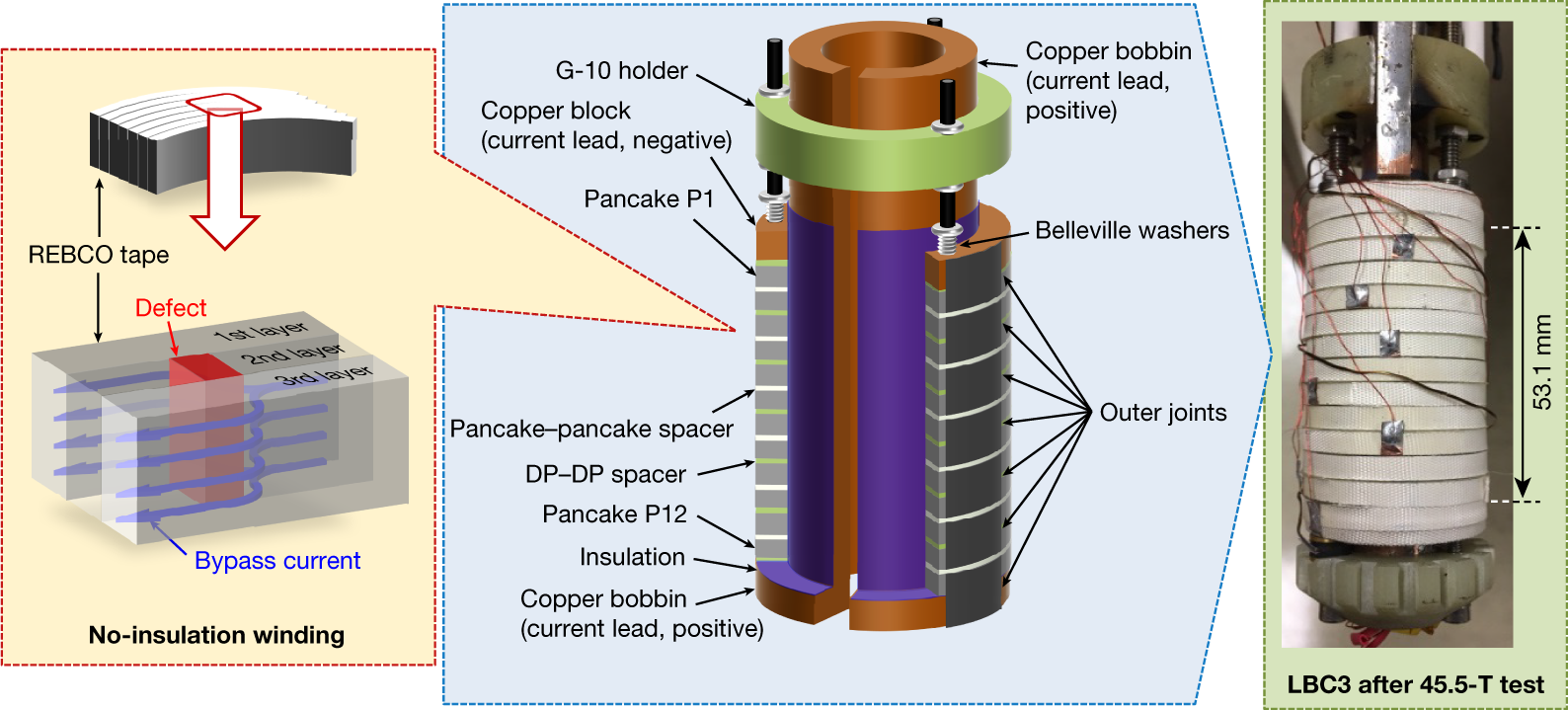
The caption:

The caption:

The caption:
Quenching the current however led to some degradation of the tapes.

The caption:
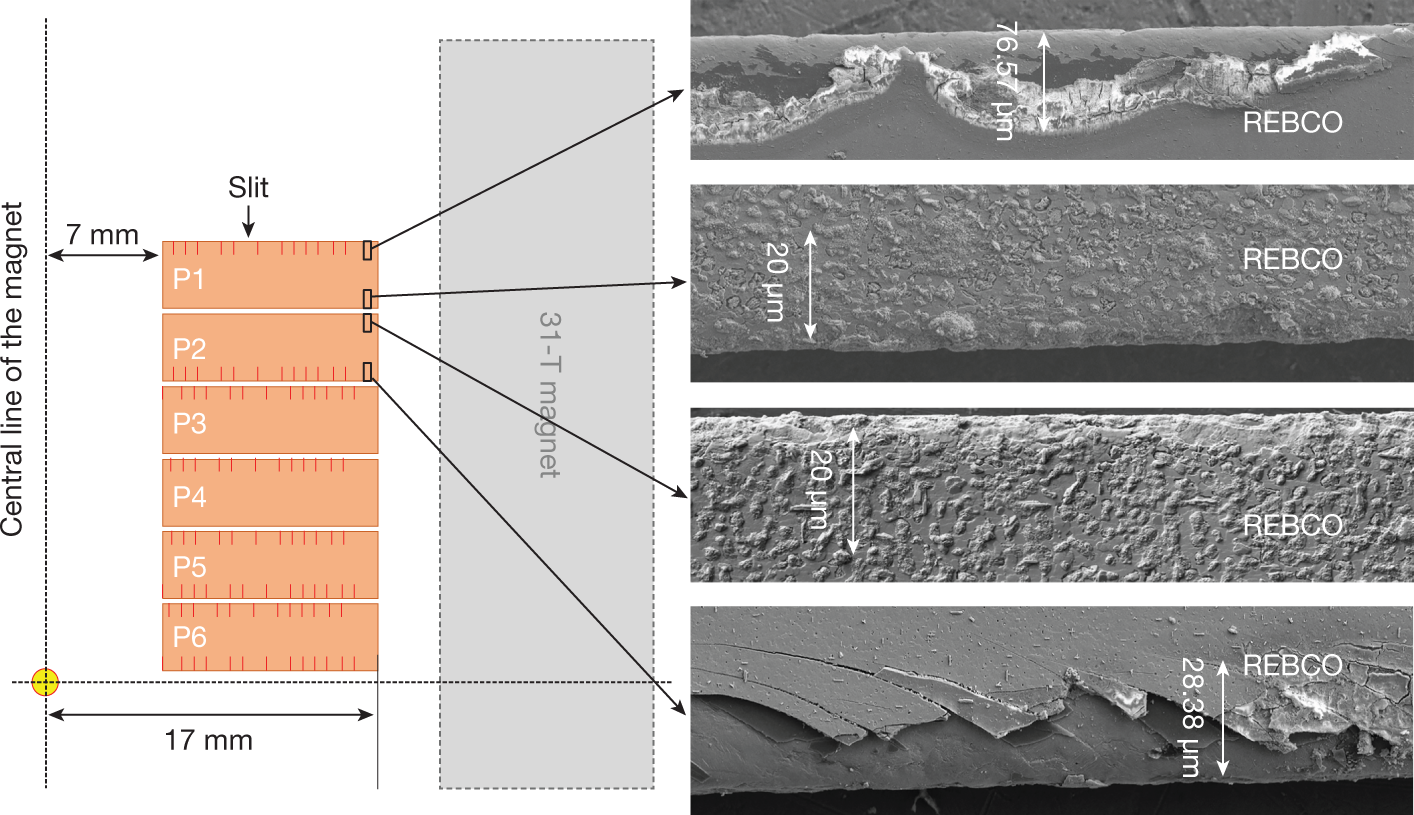
The caption:
Some concluding commentary:
Cool...or hot...
Well, you get the idea.
I trust you had a very pleasant Wednesday.

AJT
(5,240 posts)Basically, I don't understand most of what you wrote, but I am eternally grateful that people like you exist. The rest of us would be lost without you.
Thomas Hurt
(13,903 posts)NNadir
(33,515 posts)Charged particles in a magnetic field move effectively in a circular motion depending on their speed, BXv.
eppur_se_muova
(36,261 posts)... back when it was housed at the Francis Bitter Magnet Laboratory at MIT. (It was then known as the Francis Bitter National Magnet Laboratory; somebody in Congress decided that "elite" universities shouldn't monopolize National Labs, so they moved it to, um, Northeast FL, which is just crawling with the technically educated professionals you would want to hire at such an institution, unlike stuffy old Cambridge, MA.) I recall that in some projects they were using high explosives to compress magnetic field fluxes to obtain transient magnetic fields of very high strength, at the expense of destroying the sample being studied, and part of the instruments doing the studying. Haven't kept up with the highest *steady* magnetic fields achieved by more conventional means, but knew it was getting into the ridiculous range.
A visit to the Francis Bitter {not National} Magnet Laboratory Web site shows they seem to be mostly an NMR center these days. When I was there, they had two 40-ton flywheels in the basement which could supply 8 MW of DC power in two-second bursts to be released when they needed it for particularly power-sucking magnets. Unfortunately, the rumble of the wheels caused laser apparatus to vibrate noticeably even on the uppermost floors. I guess that's all moved to FL, or outmoded, now.
The folks down in FL seem to have taken over the area of magnet development that started at Bitter, and are advertising a 100 Tesla (pulsed) magnet in development. Thats one million Gauss, if you want to make it sound even more impressive. Maybe they'll start using megagauss instead of Tesla soon. (Earth's magnetic field is ~~ 1/2 Gauss, for comparison.)
(While Googling for background info, I came across a surprisingly personal account of the history of FBNML which attributes the relocation to academic infighting. It is found here: https://henrykolm.weebly.com/mit-magnetic-lab-1961-82.html )
NNadir
(33,515 posts)To be quite frank, I haven't thought all that much about magnet technology, I always got what I needed from straight up 1D NMR at around 360 MHz, and my interaction with the magnets was basically being annoyed with myself to no end when I forgot to take my wallet out of my pocket when loading the sample, which demagnetized all of my credit cards and ATM cards.
I have sort of lazily assumed that the most powerful magnets were all superconducting, and your links and discussion made me realize that I was missing quite a bit, particularly knowing about Francis Bitter.
For NMR, I can't say I've ever been particularly involved in 2D experiments like COSEY, NOESY, etc.
I'm an old guy.
I had some peripheral interest in magic angle spinning when I was in school in connection with the time scales of non-classical carbocations, but I never went in any deeper other than to say I've been there.
Last summer my son got to visit the NMR facility at the Sorbonne, where, during a tour conducted by Christel Gervais he was exposed to lots of solid state heteronuclear stuff; apparently there's a lot going on with that involved in an interesting new take on ceramics, specifically polymer derived ceramics, to manipulate these concepts to highly ordered nanostructures. He didn't get to submit any samples there, however. Apparently there's a lot of magic angle spinning stuff involved with that. (I really should read some of Dr. Gervais' papers, but there is so much to learn and so little time left in my life.)
I do attend lectures at PPPL every winter for the wonderful "Science on Saturday" series, and always one or two talks are marketing for fusion.
Although I'm a fission kind of guy; I would like to see fusion work; if only to have access to those high energy neutrons that surely can do a lot of fun things that would be useful to humanity. One fact that always troubled me about fusion was the reliance on superconducting magnets, and the requirement that we have some of the coldest stuff on earth, liquid helium, in close proximity to some of the hottest stuff on earth, a fusion plasma, with no real avenue to control the direction of those high energy neutrons.
Thus the most exciting thing in this paper in the OP for me was to read about the "high temperature" superconducting magnets, which makes superconductivity viable at liquid nitrogen temperatures.
That, I think, is a big deal; and the fusion people, I hope, have looked at this paper.
Helium is not a renewable resource, and we are going to run out of it, and although a fusion reactor would be making some, and we can get helium-3 from tritium, we're talking gram scales, not ton scales, because of the extraordinarily high energy density of fusion plasmas, even in comparison to fission nuclei.
Thanks again for the illuminating comment.