Science
Related: About this forumTuning element distribution, structure and properties by composition in high-entropy alloys.
The paper I'll discuss in this post is this one: Tuning element distribution, structure and properties by composition in high-entropy alloys (Zhu et al, Nature volume 574, pages 223227 (2019))
Recently in this space, I referred to the presence of the relatively rare (but extremely useful) element palladium, a constituent of used nuclear fuel: Palladium is a fission product. In that post I referred to use of the element in thermoelectric devices, which convert heat directly into electricity, as in famous deep space spacecraft like Voyager, New Horizons and the spectacularly successful Cassini mission. I argue that similar (more efficient) thermoelectric devices can raise the thermodynamic efficiency of nuclear plants, thus accelerating the ease of addressing climate change by the only feasible approach to do so.
As of this writing, the price of palladium is about $54,000/kg at a kg scale.
Non-radioactive palladium can be isolated only from rapidly reprocessed nuclear fuels or those that are continuously reprocessed, which is possible for fluid phased reactors, such as those with salt based fuels or (my personal favorite because of extremely high neutronic efficiency) liquid metal fuels like the LAMPRE reactor which operated in the early 1960's at Los Alamos using a plutonium/iron eutectic. (Other plutonium eutectics are known.)
Separation would involve exploiting the volatility of ruthenium tetraoxide generated from extracted metal samples, and allowing the obtained ruthenium's 106 isotope to decay and harvesting the non-radioactive palladium-106 daughter.
Older nuclear fuels will contain the radioactive palladium isotope Pd-107, which is an isotope representing low risk, since it is a low energy pure ?- with no penetrating radiation, that will be diluted by, Pd-104, Pd-105, Pd-106, Pd-108, and Pd-110. This should allow for wide use of this palladium, if and only if the stupidity of some of the less educated, i.e. ignorant, people responsible for 7 million deaths per year from dangerous fossil fuel and biomass combustion waste is rejected in an effort to save humanity from itself.
(The worst kind of ignorance is deliberate ignorance expressed with a complete lack of shame and with some force, that is, Trumpian ignorance. I have been sparing myself from engaging people here who represent exemplars of this kind of ignorance. As this is an issue on a national scale, I often reflect on a lecture I saw by the neuroscientist - and anti-gerrymandering activist - Sam Wang in which he claimed that the best way to give lies credibility is to repeat and report them while trying to discredit them. This may, in my opinion, be true; it's at least worthy of consideration. I wish our national media believed that. I'm personally as tired of hearing drooled drivel from the so called "President of the United States" as I am of hearing the drivel of anti-nukes.)
Anyway, isotopes decaying either to stable palladium and including radioactive Pd-107, constitute, in direct fission, about 21.8% of fast fission events in plutonium-239, the fast fission of plutonium being the most desirable in terms of sustainability and foreclosing all energy mining (including uranium mining) for several centuries using uranium already mined. (The similar use of thorium already mined and dumped by the lanthanide industry might extend this period for additional centuries.)
Note this neglects neutron capture reactions, which depend in turn on the capture cross sections of the isotopes in the fast spectrum, but is useful as a first approximation.
World Energy Demand as of 2017, according to the 2018 World Energy Outlook put out by the EIA - the 2019 edition should come out soon - was 584.98 exajoules. To eliminate all energy mining by plutonium utilization would require the complete fission of about 7,300 tons of plutonium per year, and produce, therefore, about 1,500 tons of palladium per year. The availability of this element in such quantities would of course reduce prices and make use of the element more available, but at current prices, just for arguments sake, the value of this palladium would be about 82 billion dollars.
As for the radioactivity, neglecting neutron capture, and also neglecting the option of separating the 106 isomer, about 14.7% of the total palladium would be radioactive palladium-107. The long half life of Pd-107, 4.5 million years, yields a fairly low specific activity, about 0.4 millicuries per gram for the pure isotope, and, representing 14.7% of the total palladium, even less, 0.075 millicuries, or about 75 microcuries. As a pure beta emitter with low energy beta (0.987 keV) in a self shielding situation, it is hard to imagine than an alloy containing this palladium would exhibit any health risk to persons using it as a structural alloy, which is what the cited paper is all about.
Note that the alloy discussed therein would represent even further dilution of any Pd-107 related radioactivity, to even more meaningless levels, with the specific activities listed above reduced by a factor of five.
From the abstract:
...These deformation mechanisms in the CrFeCoNiPd alloy, which differ markedly from those in the Cantor alloy and other face-centred cubic high-entropy alloys, are promoted by pronounced fluctuations in composition and an increase in stacking-fault energy, leading to higher yield strength without compromising strain hardening and tensile ductility. Mapping atomic-scale element distributions opens opportunities for understanding chemical structures and thus providing a basis for tuning composition and atomic configurations to obtain outstanding mechanical properties.
(The abstract is probably open sourced.)
From the full paper's introduction:
Some pictures from the paper:
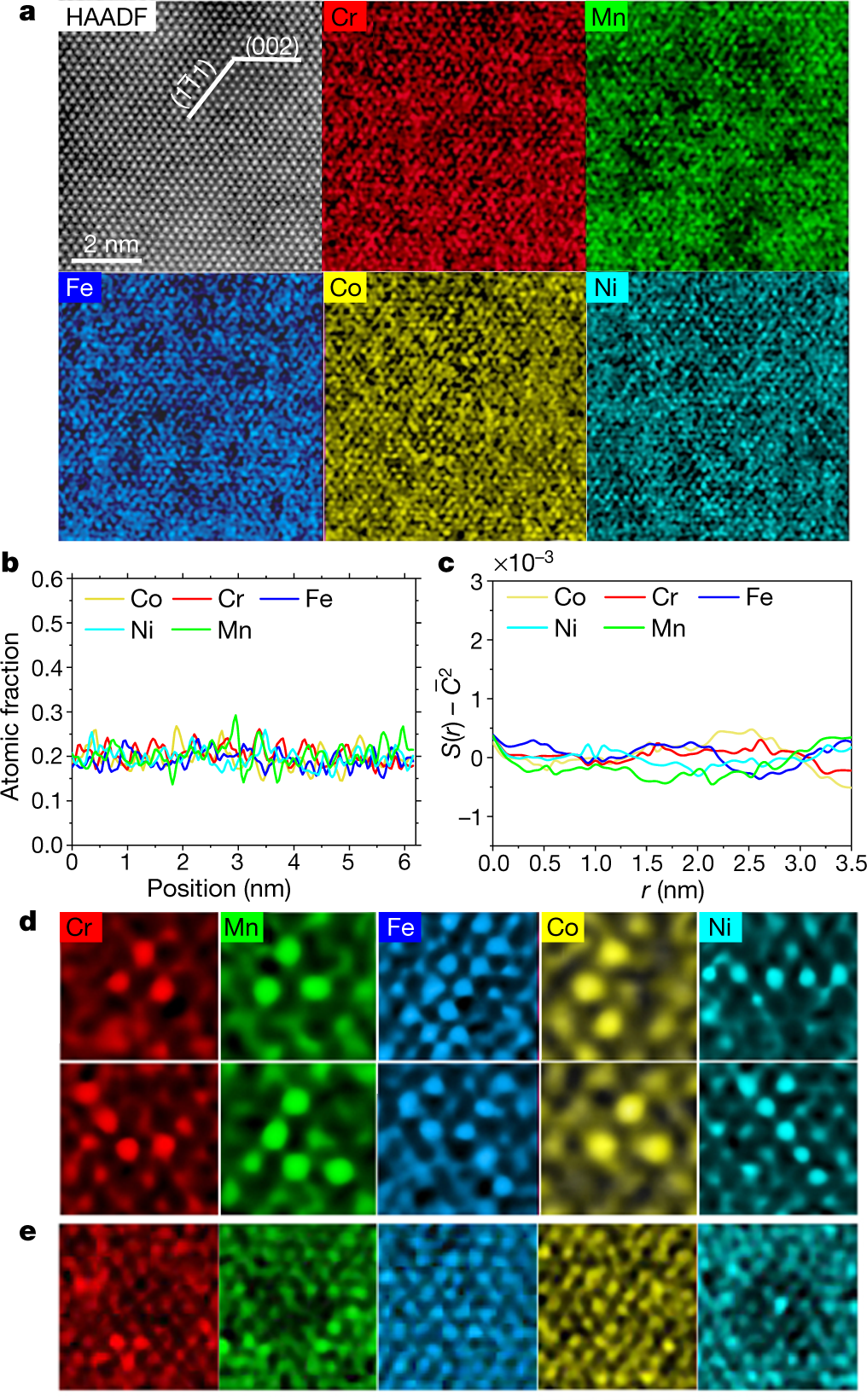
The caption:
HAADF refers to atomic-resolution high-angle annular dark field transmission electron microscopy (TEM), a techiniq my son about which probably knows the details. (I don't.) EDS refers to Electron Dispersive Spectroscopy.

The caption:
Superscripts and lines over symbols are displaced in these captions because of the limits of the DU editor, but one can get the idea.
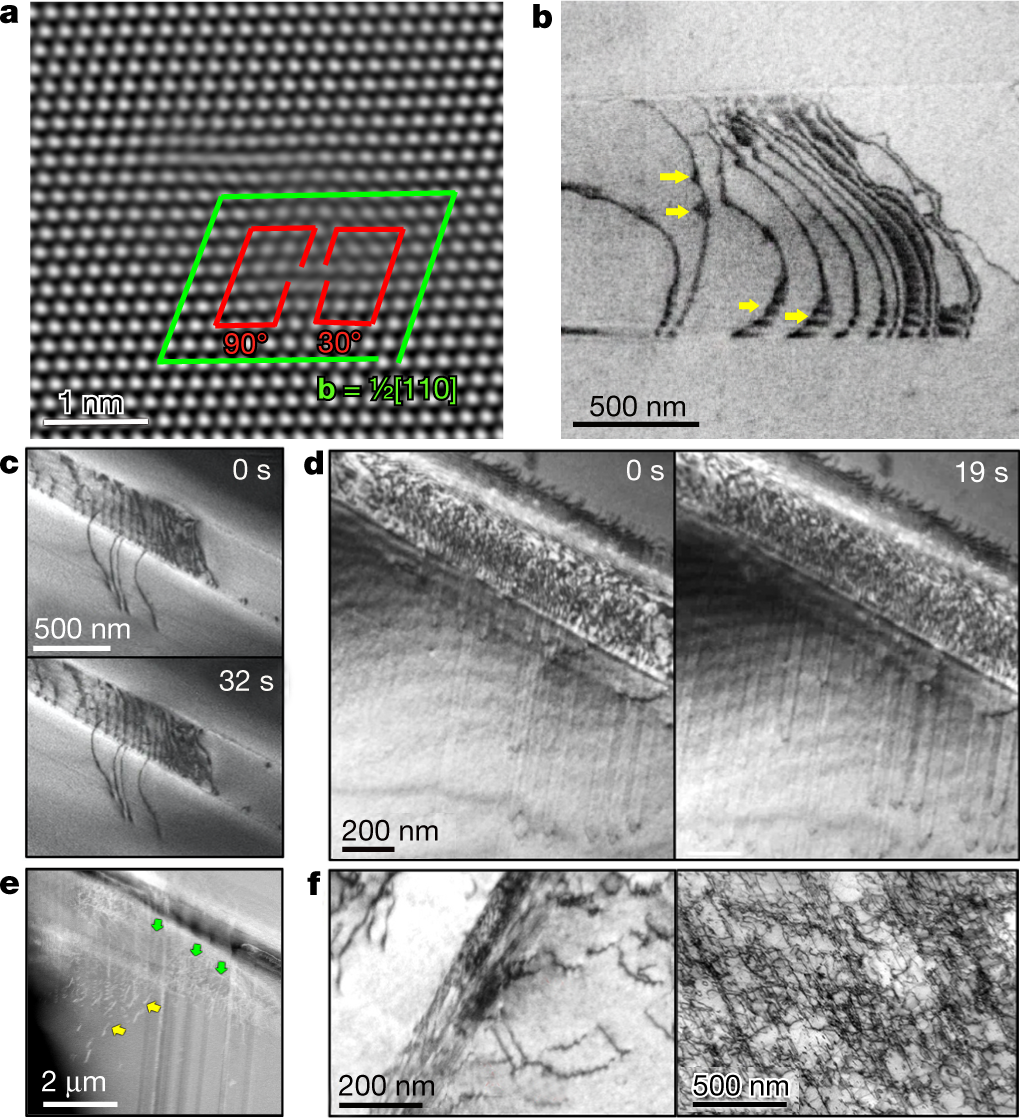
The caption:
The "money" picture:
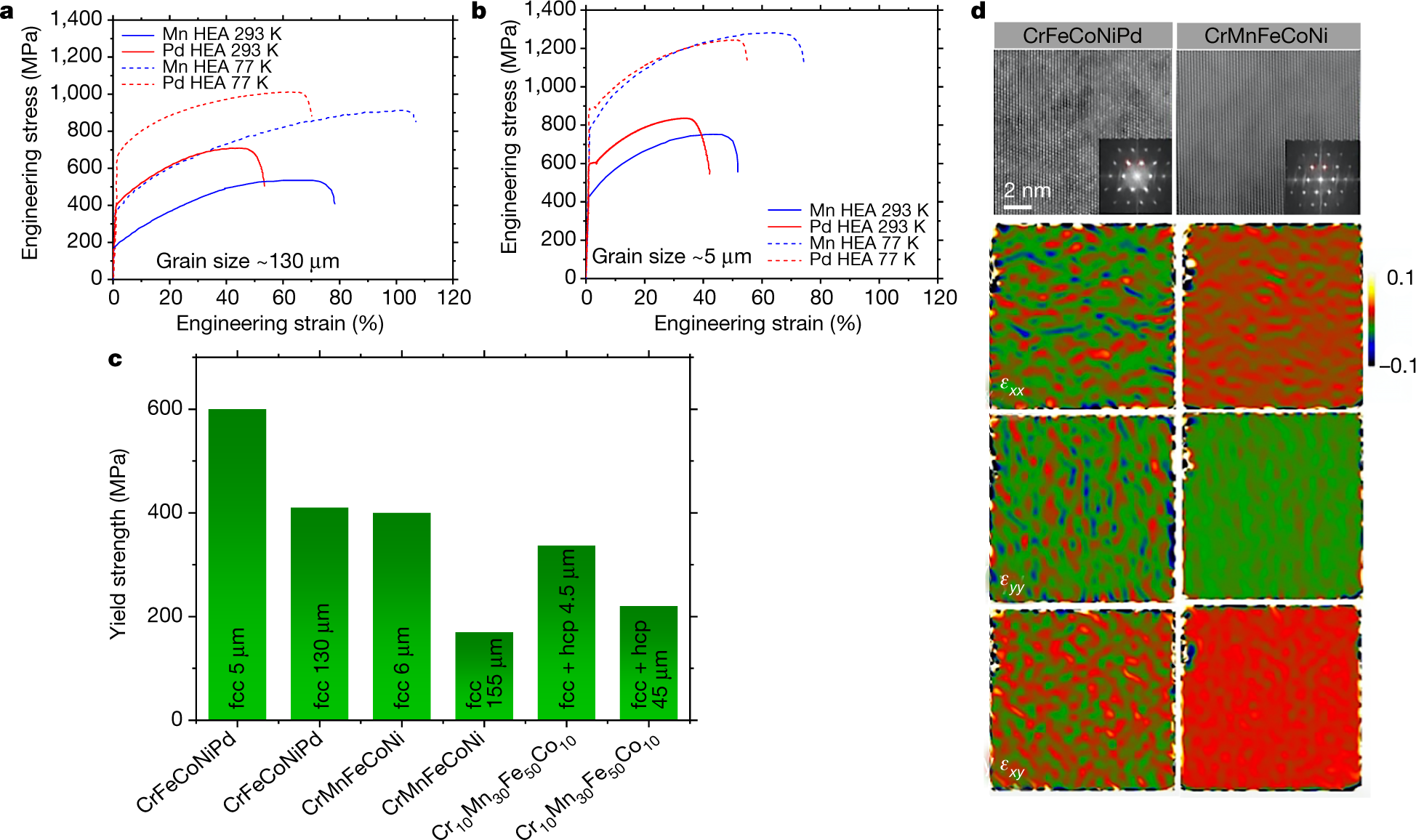
The caption:
The paper reports the temperature treatment of these alloys, but not mechanical strength at these temperatures:
Perhaps therefore with appropriate thermal barrier coatings, these alloys may be usable in high temperature turbines, where strain resistance, strength, is important. The engineering details are beyond the scope of this post, but I would like to see Brayton type nuclear heated turbines with a carbon dioxide working fluid operating at around 1400 °C for the purposes of reducing carbon dioxide. Such a system might exhibit extremely high exergy.
My son has a long weekend coming up, and will be visiting us at home. I'm looking forward to discussing this paper with him.
I wish you a pleasant day tomorrow.