NNadir
NNadir's JournalA Startling Technique: Solution Phase NMR of Live Single Cells for Metabolic Studies.
Early in my career, as I was into organic synthesis, I lived and died pretty much by NMR, simple NMR, 1D NMR.
The analytical technique most important to me these days is mass spec, and recently, as my career approaches its end, I have become aware of the use of flow cytometry which now allows for the isolation of single cells, which has many obvious biomedical applications. I am watching closely the approach of mass spectroscopic analysis.
Scientists like (among others) Vicki Wysocki at the University of Ohio and Caroline Bertozzi at Stanford have greatly advanced the mass spectrometry of biomolecules, the former in such areas as protein/protein interactions and complexes, and the latter, often mentioned as a candidate for the Nobel Prize, in diverse areas such as the very difficult area represented by the proteomic sugar code.
Mass spec, one of the most powerful analytical techniques in the world, however is inherently a destructive technique. You cannot use it on living systems.
Among the many fascinating advances in NMR of which I've learned is the ability to use it in "PAT," Process Analytical Technology - I, and some of the old folks I was with at the time - were somewhat shocked to learn that modern signal processing technology allows for the use of NMR without perdeuterated solvents.
Apparently "that ain't nothing, baby!"
I haven't touched NMR, except in an extremely peripheral fashion, for more than 25 years. Of course many people, even outside of the sciences, are aware of "MRI" "Magnetic Resonance Imaging" (from which the word "nuclear" was stripped to address the fear and assertions of ignorance the word inspires) for the imaging of biological tissues, but today in an issue of Chemical Reviews I learned that people are currently working on the single cell, live, as in living, NMR.
The paper that blows my mind:
Radio Signals from Live Cells: The Coming of Age of In-Cell Solution NMR Enrico Luchinat, Matteo Cremonini, and Lucia Banci, Chemical Reviews 2022 122 (10), 9267-9306.
Some excerpts from the introductory paragraphs:
...NMR spectroscopy is the only one able to obtain information on the structure, the kinetics, and the thermodynamics of biological macromolecules at the atomic level, as it can observe them in native-like environments at physiological temperatures, and it can do so in a nondestructive manner. (6) Such a feature has always made NMR spectroscopy appealing for the study of small and large molecules not only in vitro, isolated from their physiological context, but directly inside intact living cells. Compared to other spectroscopic techniques, NMR suffers from an intrinsically low sensitivity; therefore, its applicability to cells was traditionally restricted to the observation of small, highly abundant molecules. Indeed, in the past century, cellular NMR studies were mostly focused on the analysis of cellular metabolism, for example, by exploiting the observation of phosphorus-containing molecules through 31P NMR, or by introducing 13C-labeled precursors for a metabolic flux analysis. In some cases, very abundant small macromolecules could be studied, often because of peculiar properties that made them stand out against the rest of the milieu, as it is the case for highly shifted signals of paramagnetic metalloproteins. Then, in the early 2000s, it became clear that modern NMR spectrometers, with a higher magnetic field and more sensitive hardware, could detect signals from isotopically labeled proteins inside the bacteria in which they were recombinantly expressed. (7) Shortly after, macromolecules─proteins at first, then nucleic acids─were delivered to eukaryotic cells. The cellular NMR approach, reborn as in-cell NMR, soon gained widespread recognition, in a time when the scientific community had realized the importance of performing biochemical and biophysical studies in physiologically relevant contexts, and huge advancements were being made in developing techniques, such as single-molecule Förster resonance energy transfer (FRET) and cryo-electron tomography, that would be able to characterize macromolecules in a cellular environment...
...This work provides a detailed overview of the development and applications of in-cell solution NMR approaches during the first ?20 years since its inception in the modern sense. We first describe the existing approaches for cell sample preparation, the various types and strategies for isotopic incorporation, and the NMR methods that can be applied to living cells. We then review the application of in-cell NMR to different biological questions: how the cellular environment affects the folding thermodynamics of a protein, its structural and dynamic properties, and its interactions with specific cellular partners; whether the structure of a folded protein in cells differs from that determined in vitro...
This is unbelievable.
It's been going on for 20 years, and I missed it entirely.
At the end of my life, I feel great anxiety and shame about what my generation is leaving for future generations: They face unbelievable risks as a result of our unrealized fantasies. A saving grace, perhaps, for all the evil we have done, is that we have left for them a record of unbelievably high technology, the tools from which perhaps, they can escape the miasma of shame we have left for them.
This may be esoteric, but the implications are enormous.
Next week I'm going to see my 94 year old step mother.
It's been a long time, because of Covid, and distance.
I'm bringing my sons before my youngest moves away for graduate school.
I was in my twenties when my father, some years after my mother's death, married her, and although I wasn't looking for another "Mom," I found what a privilege it was to have one.
She is, I think, one of the most beautiful people in the world; just filled with love, selfless to the max.
I'm very excited to see her again.
Nobody has a better calcium score than I do. The doctors were all amazed. This is the best...
...calcium score they've ever seen. People are saying that they've never seen a calcium score like mine. Believe me, this is a very great calcium score. The crowds that gathered to check out my calcium score were the biggest crowds that ever gathered in the radiology department. It's a covfefe calcium score.
The degenerative changes to my spine are fake news.
The 4 mm lung nodule is the very, very, very, very best nodule. Nobody has a better nodule than mine. I know more about the nodules than the doctors do. It's a very good lung. I have the best lungs. It's natural that women are attracted to my lungs.
People are saying that I had a CT scan, and it's the best CT scan that anyone has ever had.
Sigh...
At least my doctor has the privilege of knowing he's not Trump's doctor.
Our Surreal World: K-Pop Threatens the Stability of South Korea's Economy.
Sometimes I think I died and was reincarnated in some bizarre alternate universe:
From the NY Times:
BTS Ponders Its Future, and South Koreas Economy Warily Takes Note
Subtitle:
In just a day, the stock price of the groups management label, HYBE, plummeted 28 percent to 139,000 won or $108 its lowest price since the company went public nearly two years ago, shedding $1.7 billion in market value. The stock price has barely moved since.
The drastic plunge underscores how South Koreas best-selling boy band has become not only a cultural sensation but also a powerful stakeholder in South Koreas economy.
Since the groups debut in 2013, BTS has raked in billions of dollars through album sales, concert tickets and social media. Its YouTube channel alone, which is the 20th largest in the world, can generate up to $2 million a month. By 2020, the group was contributing $3.5 billion annually to the nations economy, according to the Hyundai Research Institute...
My kid's boss asked him to do some work. My kid asked for $20/hr. His boss objected strongly.
"$20/hr, are you kidding? It's worth at least $40/hr, and that's what we're paying you."
My kid - he's no kid, he's 27 - is taking the summer off, and his boss asked him to come in and do some extra work fixing computer graphics.
My "kid" is wimpy. For some reason, I can't get it through his head how talented, and valuable, he is. It's been going on since he was small.
They gave him a "free" studio, and I think it's just because they just want him to make art.
Again and again...it's not going away, no matter how many times we forget about it.
From my inbox 2 weeks ago, the NY Times Morning Briefing:
In the early hours after the shooting at a Tulsa medical center on Wednesday, the details were murky. Soon, it became clear that the death toll there was not going to be as nearly as high as the tolls from the recent shootings in Uvalde and Buffalo.
Four people were killed in Tulsa (in addition to the gunman), compared with 21 in Uvalde and 10 in Buffalo. But the Tulsa shooting is nonetheless horrific in its own way not only for its victims and their families but also for what it says about gun violence in the United States.
Shootings that kill multiple people are so common in this country that they often do not even make national news. They are a regular feature of American life. Tulsa has become the latest example yet another gun crime that seems almost ordinary here and yet would be extremely rare in any other country as wealthy as the U.S.
To give you a sense of how common these shootings are, were devoting the rest of the lead item of todays newsletter to a list of every documented mass shooting in which a gunman has killed at least three people in the U.S. so far this year. (The Gun Violence Archive defines a mass shooting as any in which at least four people are shot, including survivors.)...
...2022, so far
Jan. 19, Baltimore: A man who worked for a gun violence reduction program was killed in an East Baltimore neighborhood, along with two others. A fourth person was injured.
Jan. 23, Milwaukee: Five men and a woman were found shot to death at a Park West neighborhood home. The police believe the attack targeted specific people.
Jan. 23, Inglewood, Calif.: The same day, a shooting at a birthday party killed four people, including two sisters, and wounded a fifth. The shooting was gang-related, the mayor said.
Jan. 29, St. Louis: A shooting near an intersection killed three young men and wounded a fourth. Police said they had no suspects.
Feb. 5, Corsicana and Frost, Texas: A 41-year-old man murdered his mother, his stepfather, his sons and the son of his ex-girlfriend in an overnight shooting. The man later fatally shot himself.
Feb. 28, Sacramento: A man shot dead his three daughters and their chaperone at a church during a court-approved visit. The childrens mother had a restraining order against the shooter, who killed himself.
March 12, Baltimore: A shooting in Northwest Baltimore killed three men in a car and wounded a fourth.
March 19, Fayetteville, N.C.: A Saturday night shootout in a hotel parking lot killed three people and wounded another three. The shooting may have been linked to a fight between motorcycle gangs.
March 19, Norfolk, Va.: Hours later, an argument outside a bar escalated into a shooting that killed three young bystanders. One of the victims was a 25-year-old newspaper reporter whose editor called her to cover the shooting, not realizing she had been killed.
April 3, Sacramento: At least five shooters fired more than 100 rounds a block from the State Capitol, killing six people three men and three women and wounding 12. The police described the shooting as gang-related.
April 20, Duluth, Minn.: A 29-year-old man who said he suffered from mental illness killed his aunt, uncle, two young cousins and their dog in their sleep. He later killed himself.
April 21, Mountain View, Ark.: A man killed his parents, another woman and her son at two homes half a mile apart in a rural community, the police say.
April 27, Biloxi, Miss.: A 32-year-old man killed the owner of the Broadway Inn Express motel and two employees in an argument over money. He fled to a neighboring town and fatally shot a fourth person. Police later found the gunman dead, barricaded inside a convenience store.
May 8, Clarkston, Ga.: Three people were shot to death and three others were wounded at a suburban Atlanta condo complex on a Sunday night.
May 14, Buffalo: An 18-year-old avowed white supremacist killed 10 people and wounded three more with an assault-style weapon in a live-streamed attack at a supermarket.
May 24, Uvalde, Texas: An 18-year-old gunman killed 19 students and two teachers at Robb Elementary School.
May 27, Stanwood, Mich.: A 51-year-old man allegedly killed his wife and her three young children at a home in Mecosta County before shooting himself, police said. The man remains in critical condition.
June 1, Tulsa, Okla.: A gunman killed his back surgeon, another doctor, a receptionist and a visitor at a medical building. He then killed himself.
As long as this list is, its also a very incomplete accounting of American gun violence. It doesnt include the at least 60 shootings that left three people dead but dont technically count as mass shootings (because fewer than four people were shot). It doesnt count shootings that wounded people without killing anybody, like one in Milwaukee that injured 17 people. And it leaves out the individual gun homicides and suicides that make up a majority of the gun violence that kills more than 100 Americans on an average day.
2022, so far, in the short attention time span of our culture.
The First Installation of 3D Printed Parts in Commercial Nuclear Reactors.
Personally, I have high hopes for 3D printed reactor cores, but that's far off, and won't happen in my life time.
A few months back, at Oak Ridge National Laboratories, a nuclear reactor component was printed, but I think it was just a demonstration. (The Museum at Oak Ridge, which I visited when taking my son to his internship, has an example of a 3D printed Jeep)
Now it appears a 3D printed component has been added to existing commercial reactors:
First use of 3D-printed nuclear fuel debris filters
Westinghouse created the StrongHold AM filter in close cooperation with plant operators Teollisuuden Voima Oyj (TVO) and OKG AB. It said the filters are fully manufactured through 3D printing techniques and offer enhanced capture features to prevent debris from entering the fuel assembly and potentially damaging the cladding, which could cause unplanned and expensive outages.
Additive manufacturing - or 3D printing - simplifies the manufacturing process by building a three-dimensional object from a computer-aided design model, usually by successively adding material layer by layer.
"As the first 3D-printed fuel debris filter for insertion in a nuclear power plant, the StrongHold AM marks a major milestone in our effort to further improve the boiling water reactor (BWR) fuel reliability by leveraging advances in manufacturing technology," said Carina Önneby, Westinghouse Vice President EMEA Fuel Delivery.
During a recent refuelling outage at the Olkiluoto 2 BWR, two fuel assemblies equipped with 3D-printed filters for exclusion of foreign material were loaded in the reactor.
"We can boast playing a pioneering role, even globally, in the utilisation of this new technology at nuclear power plants," said Fuel Procurement Team Leader Arttu Knuutila from TVO...
Anti-nukes have won the day, and the climate emergency has reached an unprecedented scale, but if anything can be saved or even restored, nuclear engineering will save and/or restore it.
This is exciting news.
A nice statement of the complexity of the task that went into saving our lives.
I don't know about you, but I know that my life was saved by RNA vaccines, in my case, the Moderna vaccines.
For my whole career I was skeptical of nucleic acid therapy, because of the complexity of the task. Happily, I was proved wrong.
Besides Kariko's brilliant discovery of the possibility of substituting pseudouridine for uridine in RNA - it seems obvious now, but trust me it wasn't and she deserves the Nobel Prize eventually - there was the matter of delivering hydrophilic nucleic acids by getting to pass intact through hydrophobic cell and organelle membranes.
This was not something that just "popped out" of nowhere. This was decades and decades of work, hard work, that came to fruition at just the right time.
Anyway, I came across this nice statement of the combinatorial challenge of making this work based on research into further refinements of the challenge met but still subject to improvement:
Hierarchical Self-Assembly Route to Polyplex-in-Hydrophobic-Core Micelles for Gene Delivery, Sundiata Kly, Lucas J. Andrew, Erin G. Moloney, Yuhang Huang, Jeremy E. Wulff, and Matthew G. Moffitt Chemistry of Materials 2021 33 (17), 6860-6875
The statement of the challenge that I found therein:
To serve as effective gene delivery vehicles, PNP vectors should be designed with four main characteristics. First, they should provide stable encapsulation of nucleic acids while protecting them from constituents in the extracellular environment; for example, both deoxyribonucleases (DNases) and ribonucleases (RNases) will bind and degrade exposed exogenic nucleic acids in the blood. (4,17,18) Second, PNP vectors should disperse in the bloodstream and deliver nucleic acids to target cells through passive or active targeting. (4,17,18) Third, they should facilitate cellular uptake followed by the release of nucleic acids to trigger the desired transfection events. (4,17,18) Finally, the vector material should be broken down and excreted by the patient with minimal toxicological effects. (4,17,18)
Meeting those 4 conditions in a combinatorially effective way was a huge, huge, huge triumph, unbelievable really.
As I understand it, the technology behind the Moderna and the BNT/Pfizer vaccines was not polymer based but relied on quasi-liposomic ionizable lipids incorporated into standard liposome lipids that nonetheless can be said to have engaged in self assembly, self assembly being the difficult key that underlies all life on Earth and perhaps elsewhere.
Many of us, myself included I'm sure, owe our lives to this science.
California Drought May Severely Impact Power Output This Summer.
It is rare, in most accountings, that hydroelectric power is not separated as a separate category from other forms so called "renewable energy" although it is generally superior to solar and wind inasmuch as it is generally reliable energy...until now.
Hydro is far more productive than solar and wind combined; it produces slightly more than half the energy per year as nuclear energy provides, whereas solar and wind combined produce about a third as much energy as nuclear provides:
Source: IEA World Energy Outlook, 2021, page 294, Table A1A
The failure to address climate change despite all those cool pictures of landscapes rendered into industrial parks for wind turbines, thousands of acres of land laced with solar cells, blah, blah, blah we've been looking at for this entire century has impacted water flows, as is now increasingly understood.
Power is an industry trade journal to which anyone can subscribe, as I do. An article on the availability of power at the every same time we expect extreme temperatures:
California Drought Could Severely Limit Hydropower This Summer
An excerpt:
The EIA suggests in a supplement to its May 2022released Short-Term Energy Outlook that current drought conditions in the state potentially have a significant impact on power markets throughout the West from June through September 2022. That impact could be different than in past years, given the states accelerating growth in intermittent generating capacity and reliance on imports, which accounted for nearly one-third of Californias power supply in 2020, it said.
Conditions this summer are especially precarious given that California has experienced more frequent and intense drought conditions over the past decadeand it is currently grappling with a third continuous year of drought.
During the 2022 water year, which began October 1, 2021, snowpack reached above-normal levels in December, but dry conditions then persisted through March. As of April 1, which typically marks the peak of snowpack, Californias snowpack had an equivalent water content of 6.9 inches, which is about 40% below the median value from 1991 through 2020, the EIA explained. Less snowpack means that, as temperatures warm in the spring, less snow will melt and flow into Californias reservoirs.
Drought Widely Affecting Major Reservoirs
According to the California Department of Water Resources, every reservoir in California is suffering below-average conditions. These include reservoirs where some of the states largest hydropower generators are located, such as the 644-MW Edward C. Hyatt plant at Lake Oroville; the 300-MW New Melones plant at New Melones Lake; the 714-MW Shasta plant at Shasta Lake; the 140-MW Trinity pant at Trinity Lake; the 207-MW Folsom plant at Folsom Lake; and the 315-MW Colgate plant at New Bullards Bar Reservoir. The six plants, notably, are the focus of the EIAs modeling in the May-released supplement. The six projects accounted for 22% of Californias total hydropower generation over the previous five years, the EIA notes...
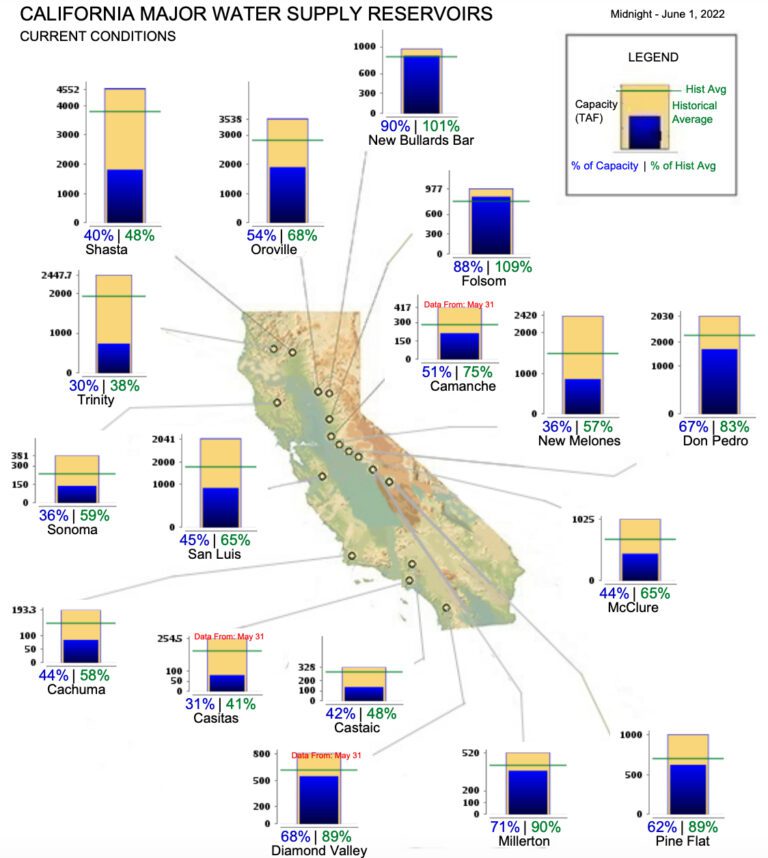
The caption:
Prices are expected to rise for power in the State; how high they rise is a function of whether the wind blows.
Today in Indio, CA, the day time high was 40.6°C (105°F). On Thursday the temperature will be 43.9°C (111°F). In the absence of air conditioning, which can take place in the absence of electricity, these kinds of temperatures can easily prove fatal.
The population of Indio is about 90,000 people.
Don't worry, be happy. We can always manufacture a few billion batteries that charge in 10 minutes and be saved.
History will not forgive us, nor should it.
Do bald face liars actually believe their lies? I have only familial experience with the sensation.
Bill Barr, trying to distance himself from his own criminality, and perhaps trying to go "easy" on the person whose corruption he served, Trump, speculated that perhaps Trump believed his own lies.
I don't think Trump is a very good liar; he really doesn't seem bright enough to do it.
I have a brother who is an expert liar, the absolute best, as well as a world class grifter. I haven't spoken to him in close to twenty years, and probably never will speak to him again.
I told my sons, that if somehow he finds out that I've died, and shows up at the funeral and introduces himself, they will think he is one of the coolest people they have ever met. They will find him to be witty, extremely intelligent, interesting, dynamic, incredibly funny and they'll be very caught up with him. I told him that before the evening is over, he will find a way to steal their underwear and then sell it back to them at a high price, whereupon they will feel like they got a bargain.
I tried to live up to my father, who I admired for all the times he forgave his brother, my uncle. I forgave my brother oodles of times, but when he was charming members of my extended family out of their money, this after I could no longer be rolled, I tried to warn them, but they told me I was being harsh, until in the end, they got rolled and understood.
Here's the thing. I, and other people, often wondered if my brother believed his own lies, he was so damned convincing and a master of the set up. On reflection however, the lies he seemed to believe always accrued to his benefit, and to the loss of everyone surrounding him.
A good liar is only a good liar if he can convince others that he believes his own lies.
Trump is a simpleton and couldn't hold a candle to my brother. (I really don't understand how Trump's grift works; it seems so transparent.) But let's be sure, that he fully understands he is lying, just as my brother did.
You have to lie to run a successful fraud; my brother's run many, but make no mistake. They always know they are lying; they just lack the ethics to care.
For what it's worth, that's my opinion, based on some close up experience.
Profile Information
Gender: MaleCurrent location: New Jersey
Member since: 2002
Number of posts: 33,518